Optimizing the mass and natural frequency of the NASA EXCITE bracket with Field Optimization
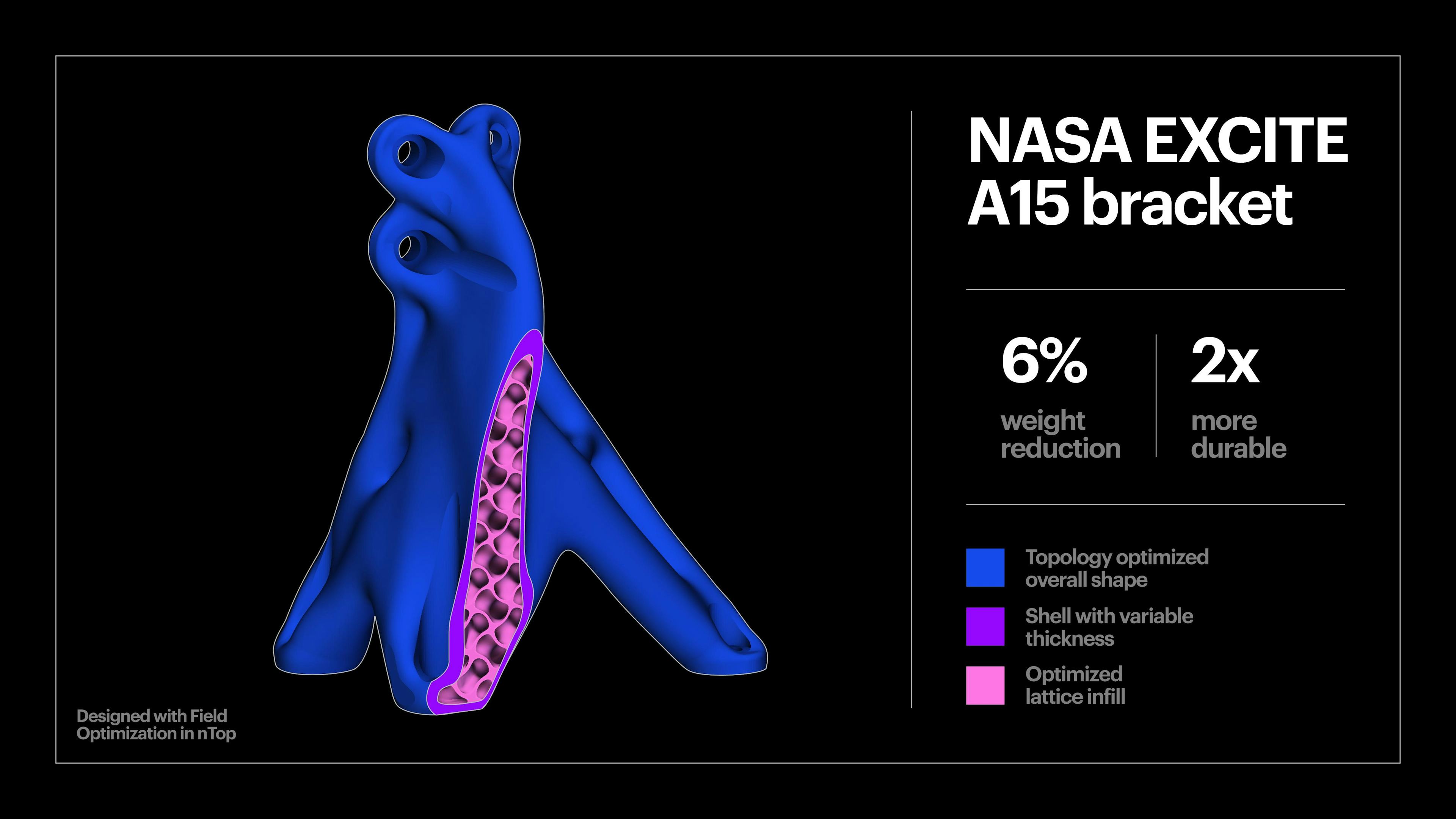
Written by Chase Allan | Associate Product Manager at nTop
Published on June 14, 2023
Applications
Key Software Capabilities
- Design automation
- Field Optimization
- Lattice structures
- Topology optimization
Aerospace engineers have used hollow structures for decades to create lightweight, stiff, and vibration-resistant parts. However, most topology optimization tools cannot easily produce hollow members.
In this article, we describe the processes we used to optimize the A15 bracket design benchmark of NASA’s EXCITE mission for additive manufacturing (AM) using Field Optimization, nTop’s new generative design technology.
Using the shell and infill optimization module of Field Optimization, in combination with traditional topology optimization, we designed a bracket that is 6% lighter than the best design alternative and drastically stiffer and stronger to vibration loads.
This project shows how we can combine additive manufacturing with the latest optimization tools to take traditional engineering concepts to the next level. Using familiar structural optimization processes, engineers can generate precise parts with a variable shell and lattice infill, leading to further weight reduction and higher performance.
What is Field Optimization?
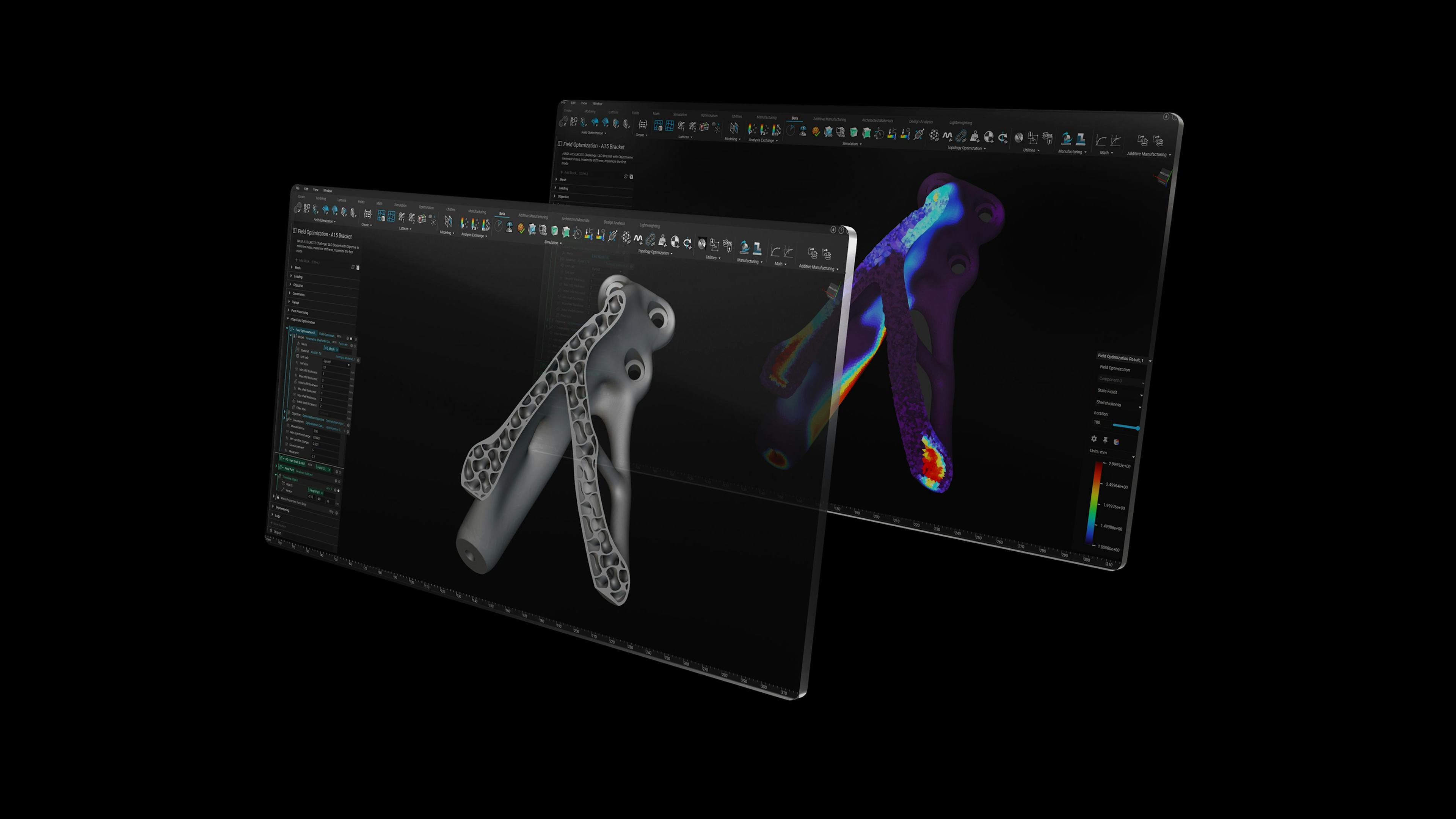
Field Optimization's shell and infill module generates optimized hollow structures that meet competing objectives and constraints in a single-step optimization process.
Field Optimization extends nTop’s Field-Driven Design and design optimization capabilities. It enables nTop users to spatially optimize an array of design parameters like shell or lattice thickness.
Field Optimization is especially valuable for parts that must fulfill multiple and competing design objectives and employ lattices or other structures that leverage the potential of AM technologies. Unlike manually iterative approaches, Field Optimization does not break down or become overly time-consuming when geometric complexity increases. By eliminating manual iterations with Field Optimization, you can innovate with the confidence that you are developing fully optimized products that do not leave any performance on the table.
The NASA EXCITE design challenge
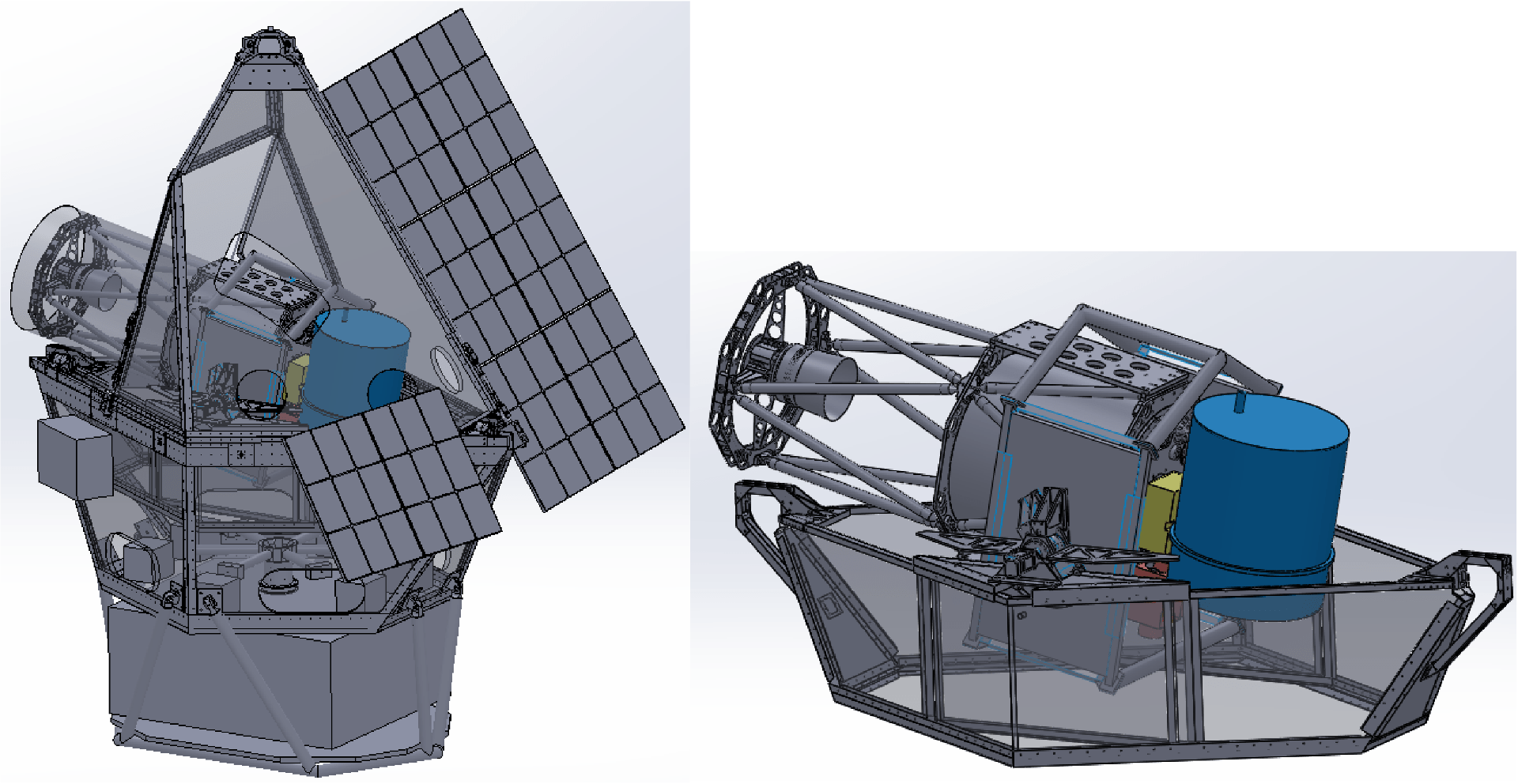
The EXoplanetClimate Infrared Telescope (EXCITE) will be a carried on a balloon and will operate at an altitude of around 40 km above most of the Earth’s atmosphere.
The design problem for the A15 benchmark comes from the NASA EXCITE mission. The bracket is used to mount the tip and tilt mirror to the back of the satellite telescope.
The goal was to minimize the bracket’s weight and make it as stiff as possible — in technical terms, maximize its natural frequency — while withstanding the forces applied during flight. Moreover, the design of the final bracket must connect to the specified bolt points and avoid areas needed for the movement of the mirror.
NASA previously presented this classic optimization problem to other teams who used different design software and traditional topology optimization tools. We used these previous results as a benchmark for the nTop shell and infill optimization workflow.
Optimization process with Field Optimization
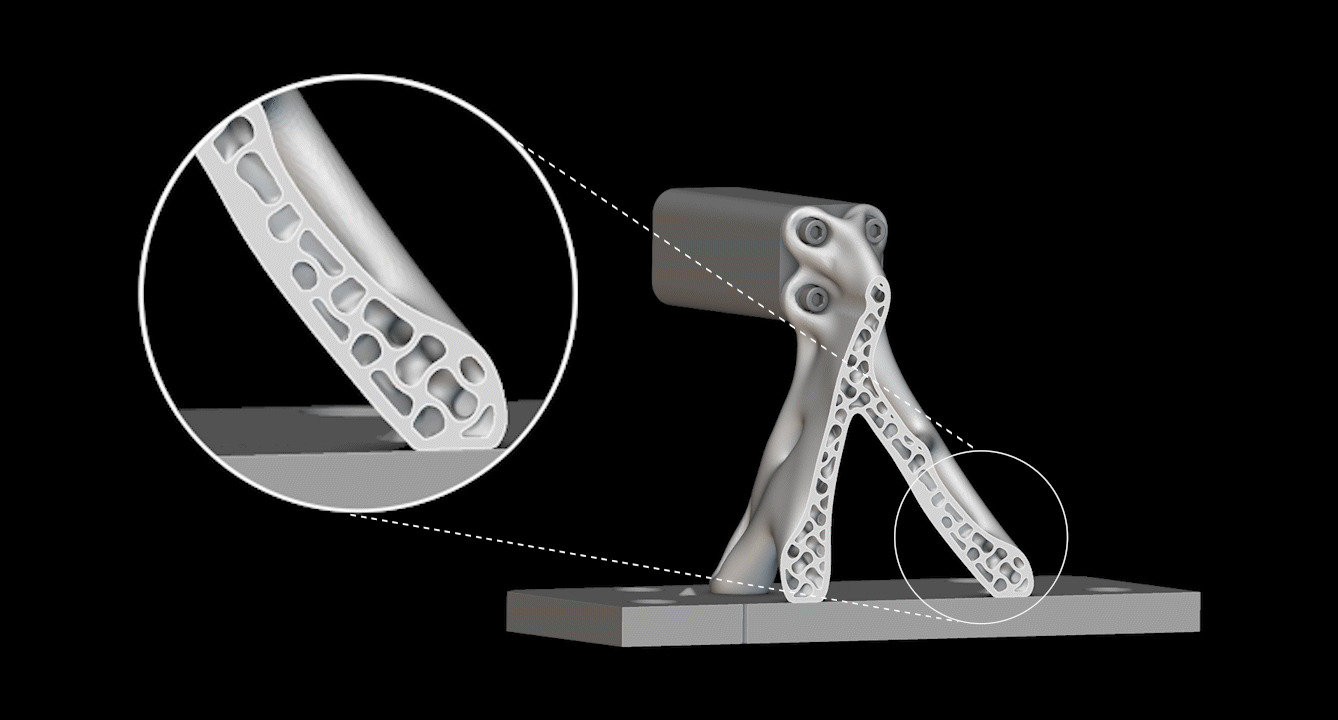
Animation of the Field Optimization iterations, starting as a homogenous shell and infill and progressively generating an optimized structure with variable thickness.
Our approach to tackling this challenge followed three steps:
First, we ran a topology optimization study to refine the design space. Since additional optimization steps would follow, we used a coarse mesh to speed up the optimization process. The objective was set to reduce weight while maximizing stiffness.
Next, we used Field Optimization's shell and infill capability using this reduced design space as a starting point and the same design objectives and constraints. We specified the minimum and maximum thickness values based on the limitations of our AM system. We opted for a gyroid TPMS infill for its good manufacturability, near-homogeneous properties, and high stiffness-to-weight ratio. This step generated a hollow part with varying shell and lattice thicknesses.
For the final steps before manufacturing, we added a powder escape hole. We also ran simulations — static and modal analyses — to validate the results and determine the structure’s natural frequencies.
Two samples were manufactured in AlSi10Mg on an EOS M290 AM system. To send the part to print, we used nTop’s Implicit Interop capability to export the design directly as an nTop Implicit File to EOSPRINT for slicing without meshing. Bypassing the meshing step allowed us to preserve geometric accuracy and avoid heavy meshing computations.
Lighter and stronger aerospace structures
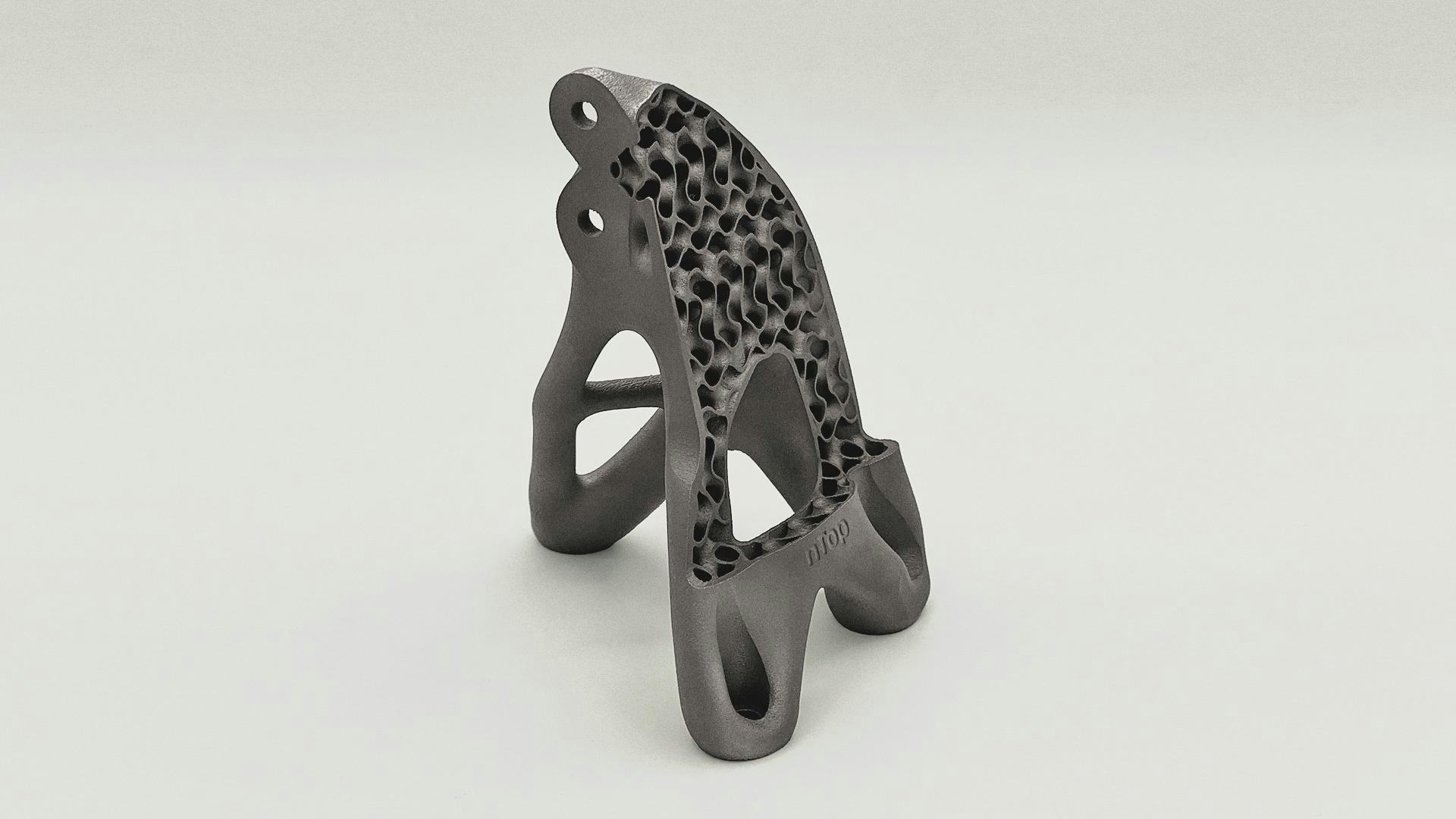
The A15 bracket was manufactured in AlSi10Mg on an EOS M290 machine.
Following this process, we generated a design that outperformed past solutions in every key performance requirement set by NASA. The final design of the A15 bracket was:
- 6% lighter than other designs, weighing approximately 188 grams.
- Stiffer and stronger under vibration with more than double the first (non-zero) natural frequency.
The results demonstrate how Field Optimization can help engineers develop lighter, better-performing structural components. In fact, generating these fully optimized and variable-thickness shell and infill structures is impossible with any other tools.
Without Field Optimization, engineers would have to work iteratively, running multiple simulation steps to determine the optimal wall thicknesses without guaranteed success. Field Optimization now closes the loop and empowers designers to achieve better results in less time.
Additionally, this project shows how to combine traditional engineering knowledge and intuition with the latest design and manufacturing tools. Textbooks can tell you hollow structures have high moments of inertia and stiffness-to-weight ratio. What textbooks or design handbooks won’t explain, however, is how to design the infill or vary the wall thickness for optimal performance. Combining traditional engineering knowledge with Field Optimization can and, in this case, did result in a higher-performing design.
Field Optimization beyond lightweighting
In addition to the shell and infill module, Field Optimization currently features lattice optimization and Voronoi optimization capabilities that can be used in applications across industries, including medical implants and consumer products.
Moreover, Field Optimization’s open architecture enables you to build custom optimization tools that encode your expert knowledge. For example, you can expand the functionality of Field Optimization by integrating custom unit cells or material test data.
As the capabilities of nTop improve, so will Field Optimization. We are exploring additional functionalities for future Field Optimization workflows, such as thermal and thermomechanical optimization, and optimization for traditional manufacturing processes like machining, casting, and molding.
Key takeaways
- The NASA EXCITE A15 bracket was lighter than the traditional topology optimization benchmark and had more than double the stiffness.
- With Field Optimization, you can generate optimized hollowed parts and lattice structures of variable thickness.
- Field Optimization’s open architecture and future capabilities make it a valuable tool for advanced engineering design beyond structural applications.
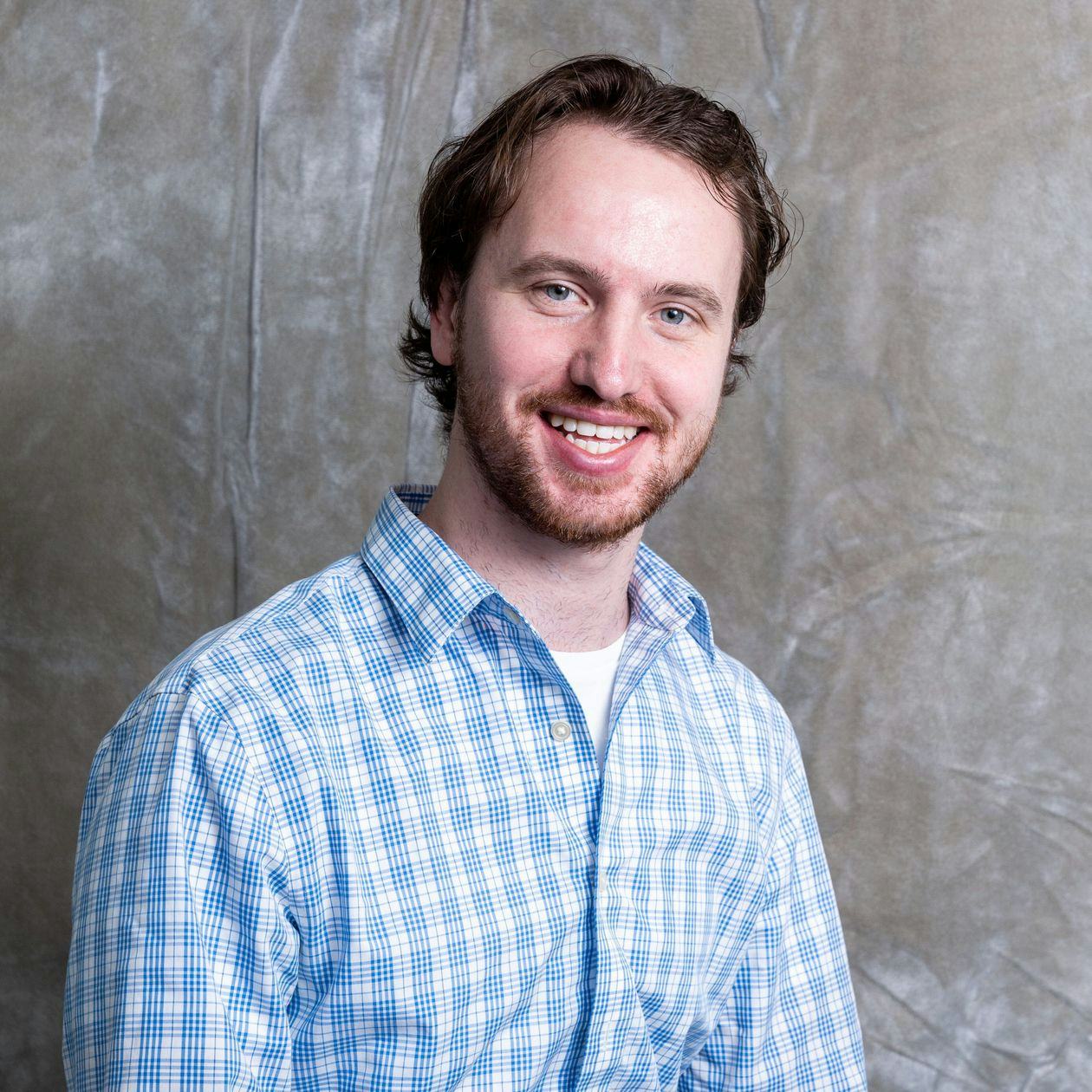
Chase Allan
Associate Product Manager at nTop
Related content
- VIDEO
Five ways to lightweight in nTop
- VIDEO
Topology Optimization Design for Cast and Injection-Molded Parts
- VIDEO
Lightweighting an impeller for additive manufacturing
- GUIDE
Download: Advanced design software and additive manufacturing for personalized implants
- ARTICLE
Optimizing thermal management with conformal cooling to extend operational life